Communication - Navigation - Weather monitoring - National defense - Entertainment
We all use and depend on these and other space-based resources, but they are vulnerable to the space environment. By studying our local plasma environment, we continue to close the gap in understanding how our place in space is impacted by the Sun.
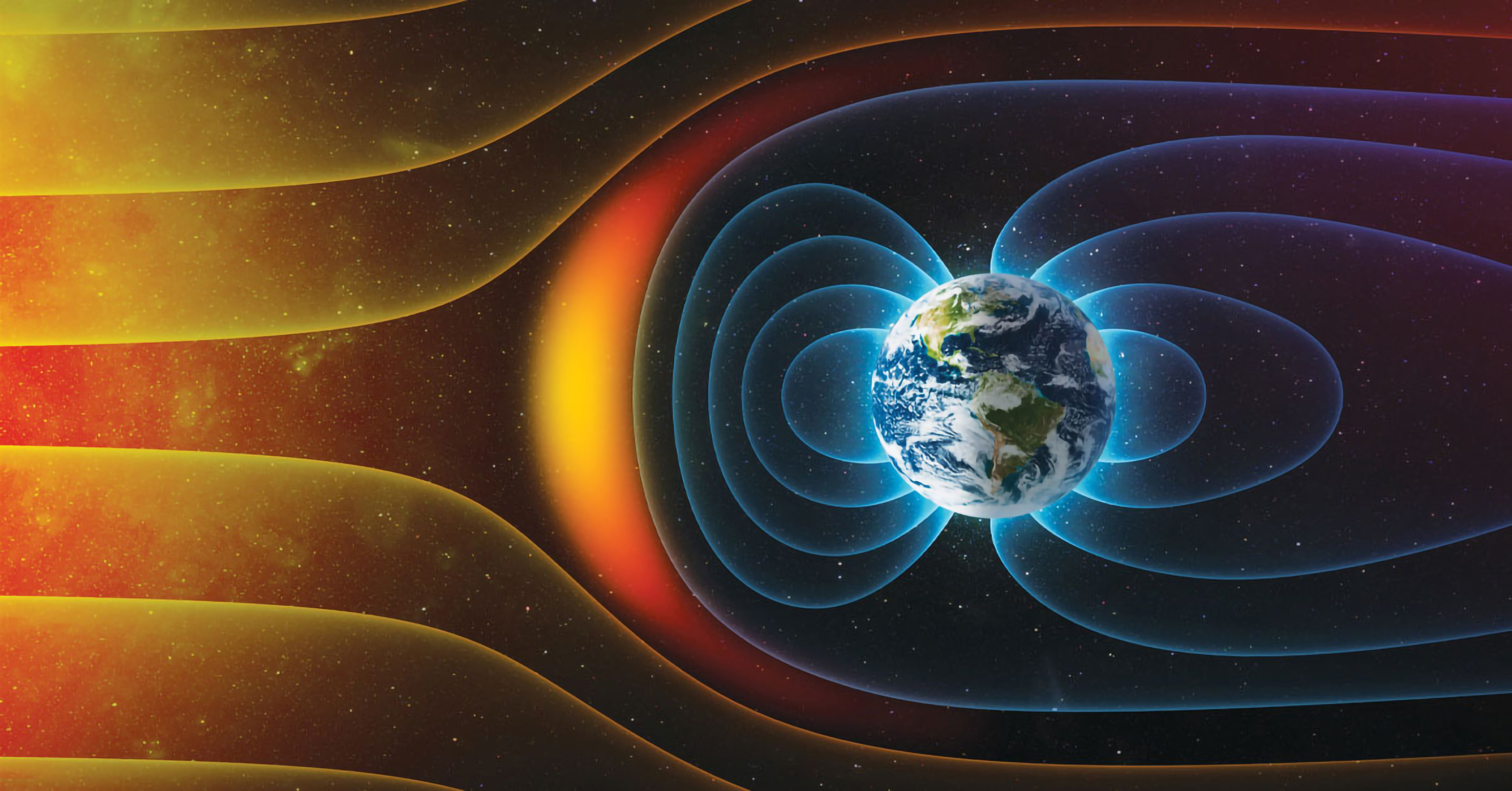
Credit: NASA
Where, how, and why the solar wind couples to near-Earth space is a major unresolved scientific question at the heart of the TRACERS mission. With its two satellites traveling in tandem, TRACERS will take us a long way toward answering this and related questions.
TRACERS overarching goal
Connecting the magnetospheric cusp to the magnetopause - discovering how spatial or temporal variations in magnetic reconnection drive cusp dynamics.
To address the overarching goal, TRACERS has three major scientific objectives
Determine whether magnetopause reconnection is primarily spatially or temporally variable for a range of solar wind conditions.
For temporally varying reconnection, determine how the reconnection rate evolves.
Determine to what extent dynamic structures in the cusp are associated with temporal versus spatial reconnection.
Helpful information
The Magnetosphere
is the region of space around the Earth that is influenced by its magnetic field. The magnetosphere is shaped by the interaction between the Earth’s magnetic field and the solar wind, which is a stream of charged particles (ions and electrons) emitted by the sun.
The Cusp
is a specific region located near the north and south poles where solar wind particles can enter the magnetosphere. It is an important area for studying energy transfer and particle acceleration processes.
The Magnetopause
is the boundary between the Earth’s magnetosphere and the solar wind.
Magnetopause Reconnection
occurs when the magnetic fields of the Earth and the solar wind interact and reconnect, allowing the transfer of energy and charged particles between the two regions. This process plays a crucial role in the dynamics of the magnetosphere and is believed to be responsible for various phenomena, including the auroras.
Spatial and Temporal Variations
can arise due to the ever-changing nature of the solar wind and the dynamic behavior of the magnetosphere. We know reconnection occurs, but we are not yet sure in what ways it changes.
-
Spatial variations refer to changes in magnetopause reconnection that occur across different locations.
-
Temporal variations, on the other hand, pertain to changes in reconnection that happen over time.
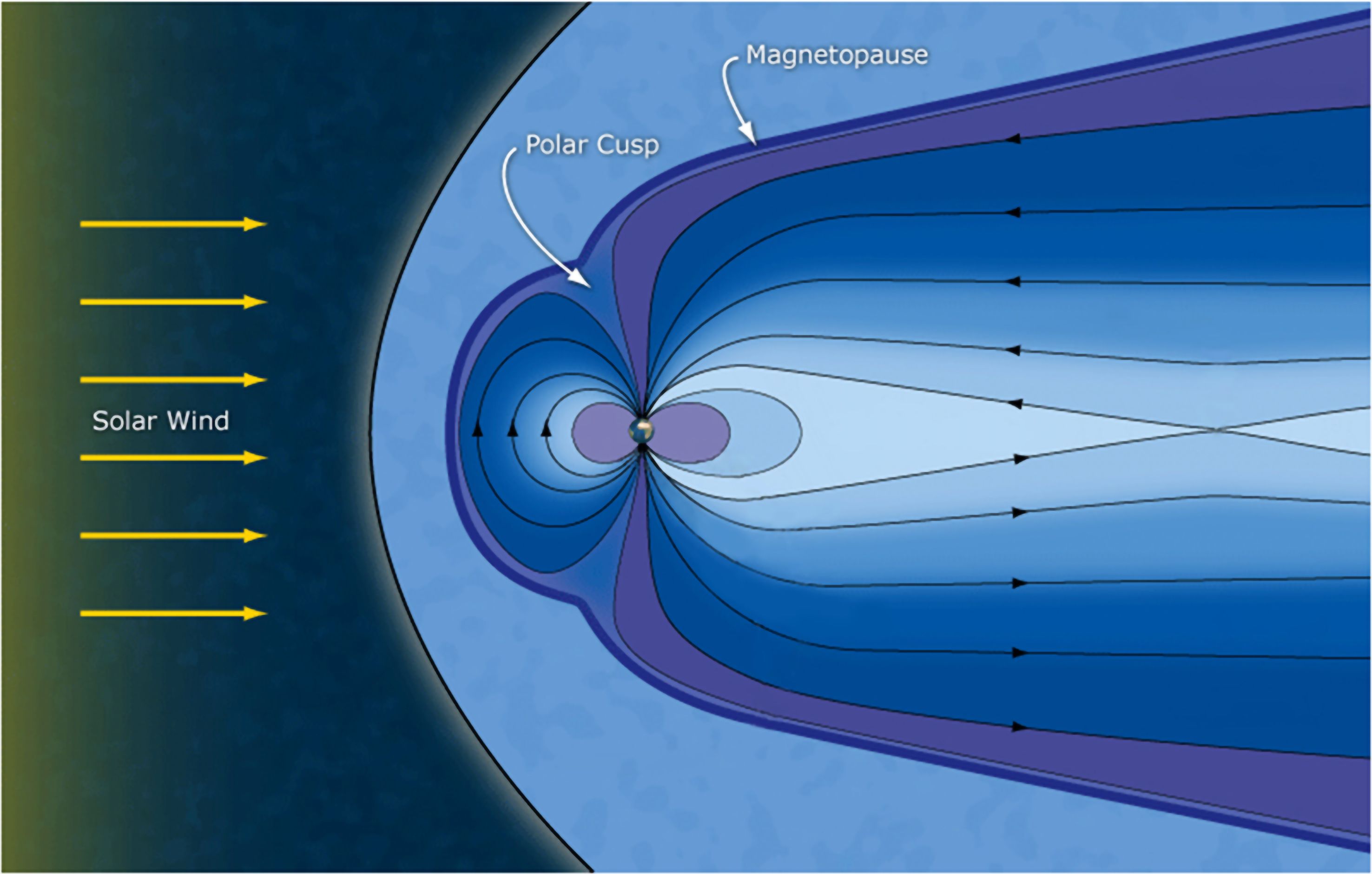
Magnetic reconnection explained
Plasma is a distinct form of matter that, although it comprises more than 99% of the visible universe, is not something most people regularly deal with. In short, plasma is formed when gas is energized to the point where electrons are ripped away from atoms, forming an ionized gas. Many things we recognize are made up of this ionized gas we call plasma: stars, nebulas, lightning, neon signs, and more.
Because plasma is high-energy by nature, it plays an important part in energy conversion processes occurring throughout the universe. One of these is known as magnetic reconnection. Magnetic reconnection is a fundamental process where magnetic energy is converted into particle energy; it is how the Sun’s solar wind connects to Earth’s magnetic field. This process occurs at the magnetopause as well as at the magnetotail toward the 'back' of the magnetosphere.
A simple description of this process: the solar wind plasma from the Sun pushes against the plasma in the Earth's magnetosphere; the magnetic field lines of the Sun and Earth bend, break, and then connect to one another (reconnect); the solar wind pushes these newly connected Sun-Earth magnetic lines back around Earth where they compress and reconnect at the magnetotail. Particles (electrons and ions) are sent toward the poles due to energy from the bending of the magnetic fields. In short, these reconnections provide a conduit for solar wind particles and energy to enter the Earth's magnetosphere through the cusp. The energy from magnetic reconnection dissipates in our upper atmosphere as auroras and magnetic storms.
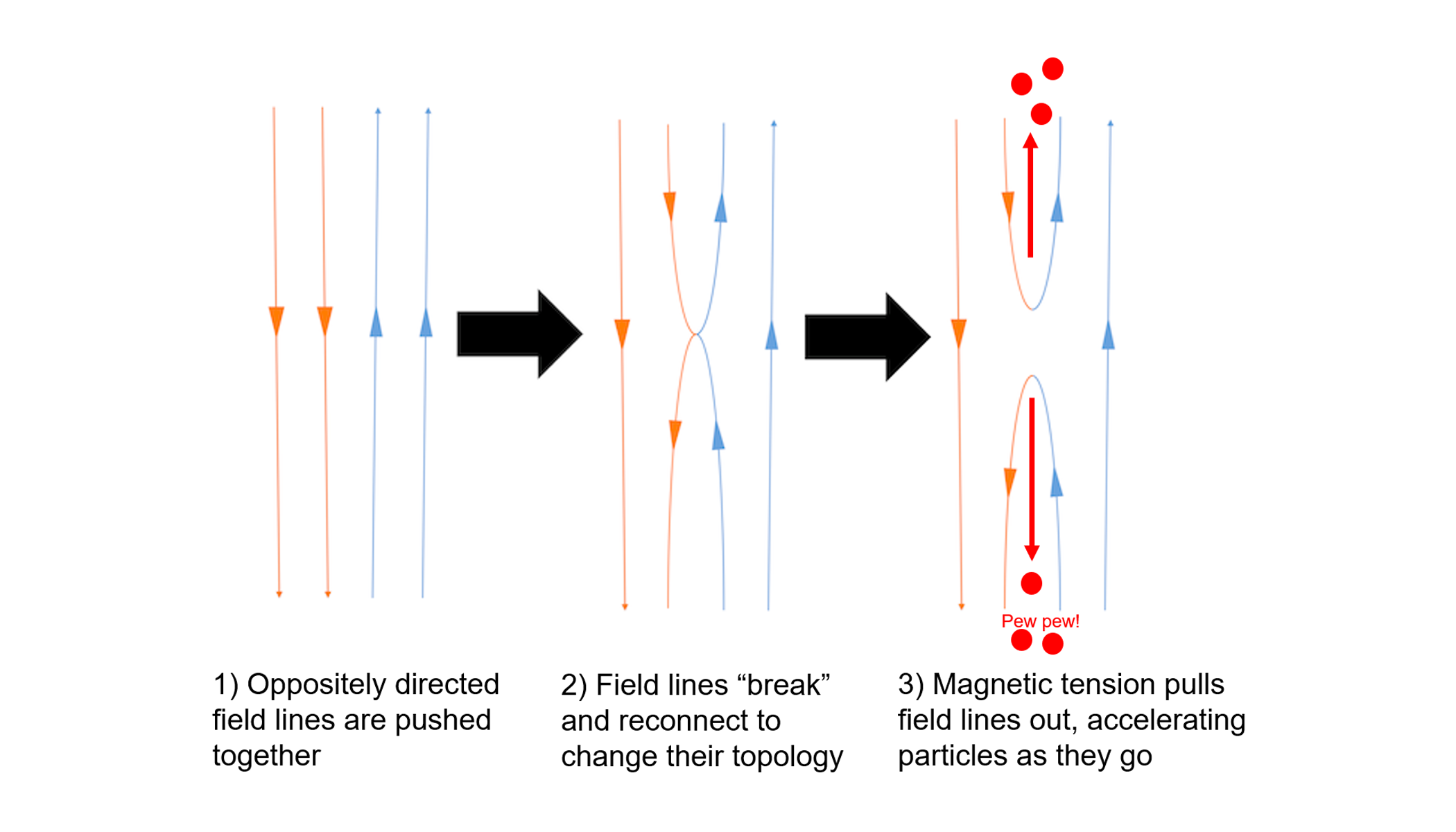
These complex processes are important to understand because, even beyond expanding our knowledge of the universe, they can impact our planet and our lives. In the 1980s, there was a large-scale power outage in Quebec, Canada. This was attributed to a relatively strong solar storm, or CME (Coronal Mass Ejection), that happened to provide Earth a 'glancing blow'. Nowadays, we are even more reliant on space-based resources and our electrical grids. The impacts of a direct hit from a stronger CME could be far worse than we have yet experienced. The TRACERS mission will help us understand, and be better able to plan and protect ourselves in the future from, some of the Sun's more destructive effects.
Animation credit: Andy Kale
Learn about the mission and magnetic reconnection from the late Professor Craig Kletzing, the original Principal Investigator for TRACERS
More on the TRACERS science objectives
Similar to how environmental conditions on Earth (temperature, moisture, air pressure, etc.) lead to a variety of weather outcomes (rain, wind, dry heat, snow, etc.), the same is expected to be true for space weather; different characteristics of the solar wind and near-Earth environment are likely lead to differences in magnetopause reconnection.
To address the mission's science objectives, TRACERS data will be used to study the interplay between the reconnection processes and the behavior of the cusp. This will help us learn what solar wind conditions lead to temporal variations in reconnection, what conditions lead to spatial variations, what dynamic structures are involved, and more.
Objective 1. Determine whether magnetopause reconnection is primarily spatially or temporally variable for a range of solar wind conditions.
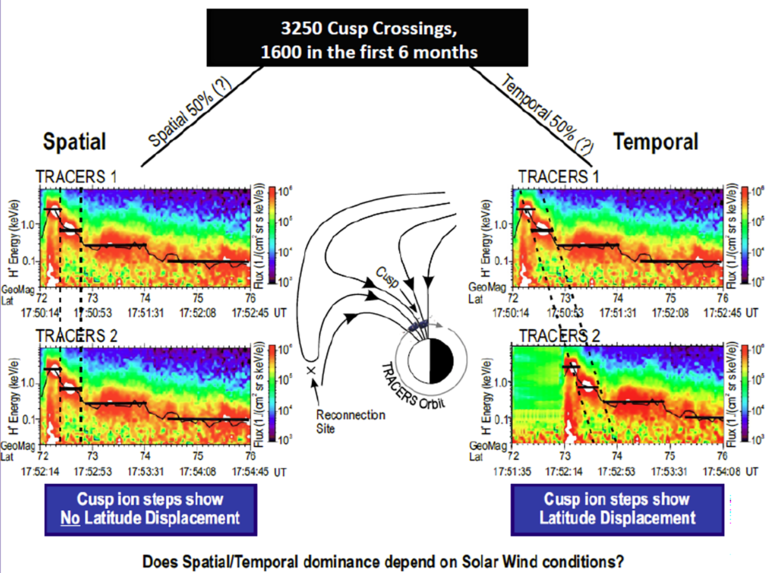
Objective 1 is the central element of TRACERS science. Researchers recognized in the 1990's that the funnel-shaped cusp provided a window into the magnetic reconnection process at the magnetopause. Although this process is occurring far from Earth (about 60,000 km!), magnetopause reconnection accelerates the ions and electrons down the magnetic field lines to the cusp.
Each time one of the TRACERS satellites flies through the cusp, we get a snapshot of this process. TRACERS is unique in that there will be two satellites flying through the cusp in rapid succession (a minimum separation of 10 seconds). What we want to know is how that snapshot of the reconnection process changes from one 'picture' to the next. We know of two possible changes.
The first is shown on the left-hand side of Figure 1. Here, we see a model of the ion measurements from the two spacecraft. In this model, nothing changes from one snapshot to the next, meaning the structure seen in the ion measurements is static in time. Therefore, the structure must be the result of spatial variations and we conclude that reconnection is spatially variable. On the right-hand side of the same figure, we see a model where the structure is the same, but moves in latitude from one snapshot to the next. This means the ion measurements were variable in time and we conclude that reconnection is temporally variable.
To address Objective 1, the ion measurements from each trip through the cusp are sorted into these categories of 'spatially' or 'temporally' variable reconnection. We then look at the solar wind conditions (in particular the orientation of the solar wind, or interplanetary magnetic field) for each of these cusp passes to determine whether spatially or temporally variable reconnection is dominant for a particular class of solar wind conditions.
Objective 2. For temporally varying reconnection, determine how the reconnection rate evolves.
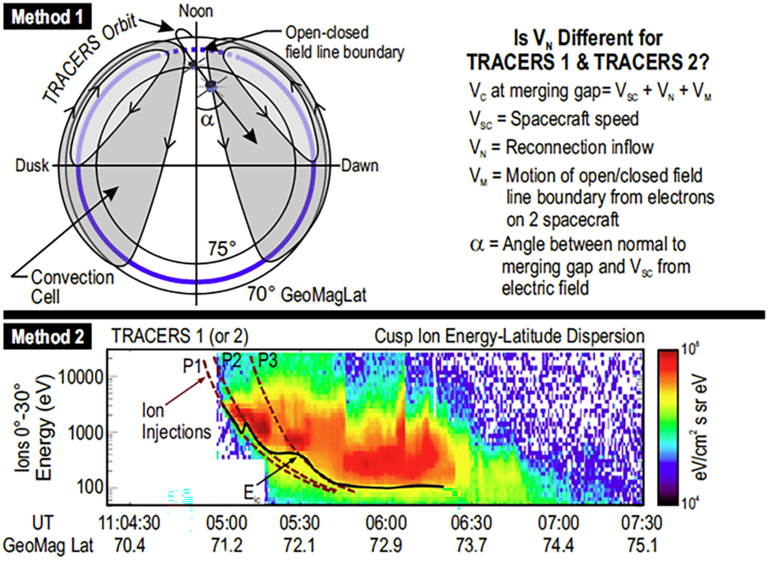
In those circumstances where magnetopause reconnection is found to be temporally variable, we also want to know how the rate of reconnection evolves (how fast the plasma is flowing into the reconnection region at the magnetopause). There are two methods for determining this.
Method 1 is relatively simple. The open-closed field line boundary or the 'merging gap' in Figure 2 is a very special place. By measuring how fast field lines are convecting through this 'gap', we get a snapshot of the reconnection rate from each of the two TRACERS spacecraft. Now, to see how the reconnection rate is evolving, we simply compare the rates found by each satellite.
Method 2 is a little more complicated. In Figure 2, we can deconvolve the energy-time profile of the ion fluxes into a series of ion injections. Each of these ion injections represent a change in the reconnection rate. Therefore, by tracing the lower edge of the ion flux (the Eic, or energy of the ion cutoff) we get a measure of the change in the reconnection rate. Figure 2 shows three injections: P1, P2, and P3. During these injections, the reconnection rate was “high”. Between them the reconnection rate was “low”. By looking more closely at the ion flux profile, we can quantify “high” and “low”.
In the past, it has been argued that the rate goes from zero to 100% and then back to zero. The problem with previous observations is that they are almost exclusively dependent on one snapshot (a single spacecraft). Therefore, it could not be determined if the rate was really varying by these large amounts or if it was simply spatial variation at the magnetopause. TRACERS, with two spacecraft providing two 'snapshots' on each trip through the cusp, will allow for a more rigorous analysis of how the reconnection rate truly evolves.
Objective 3. Determine to what extent dynamic structures in the cusp are associated with temporal versus spatial reconnection.
This objective covers the “Cusp Electrodynamics” part of the TRACERS acronym. Simply put, the Earth’s magnetosphere, ionosphere, and atmosphere are not just passive recipients of the energy generated by magnetic reconnection. In objective 1, we said that solar wind ions travel down the magnetic field lines into the cusp. However, ions are not the only things traveling along the field lines. In particular, waves follow the field lines and affect the ions and electrons. Further, the energy that is deposited into the ionosphere causes a response and an 'upwelling' of ions from the ionosphere into the cusp. What Objective 3 does is use the results from Objective 1 to help sort these phenomena into those associated with temporal variation and those associated with spatial variation of reconnection. The phenomena are complicated, but we are sure that being able to sort the phenomena will help us understand them.
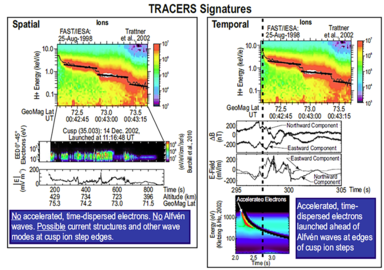
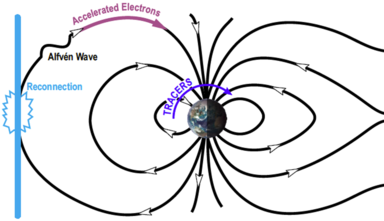
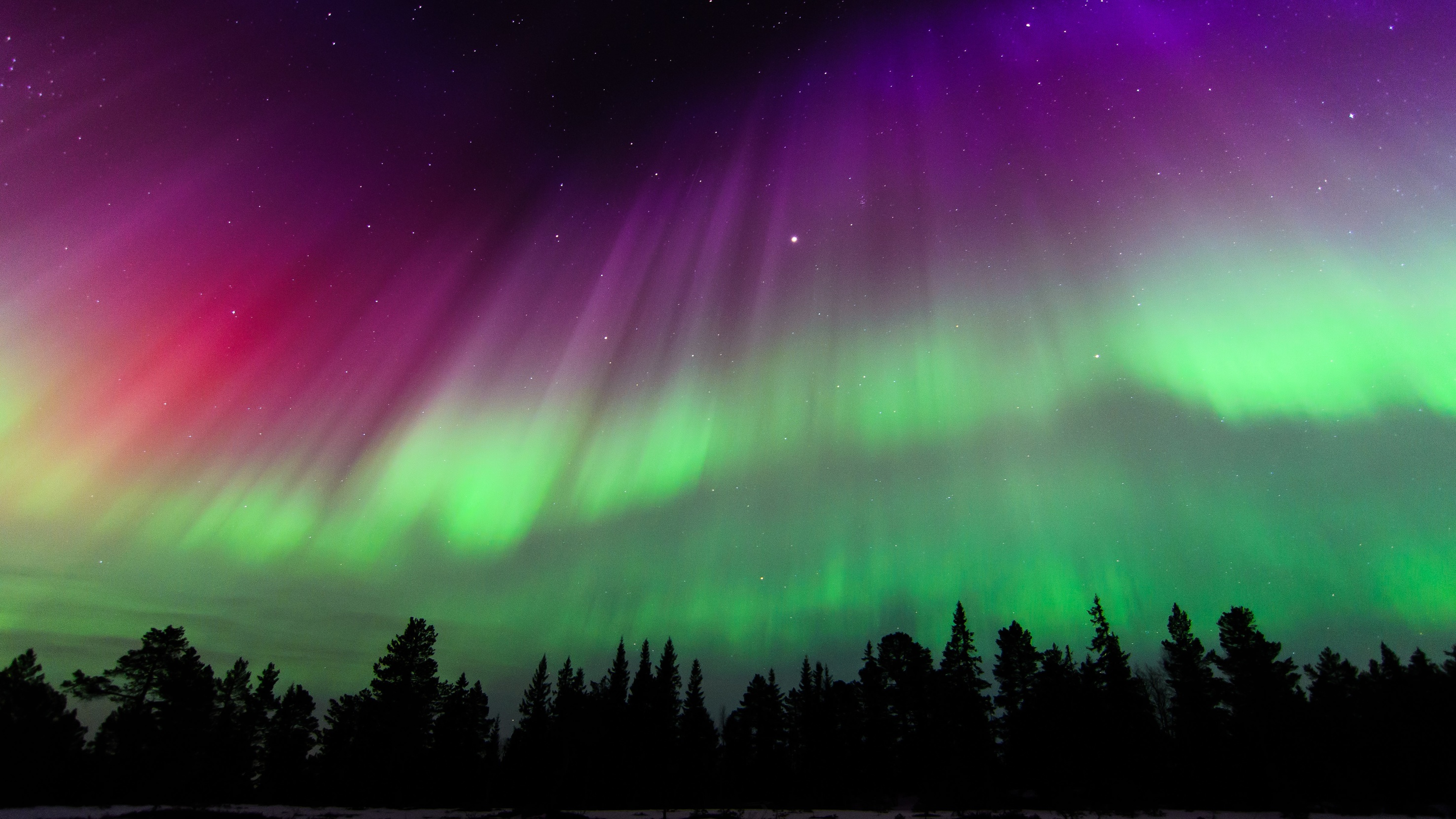
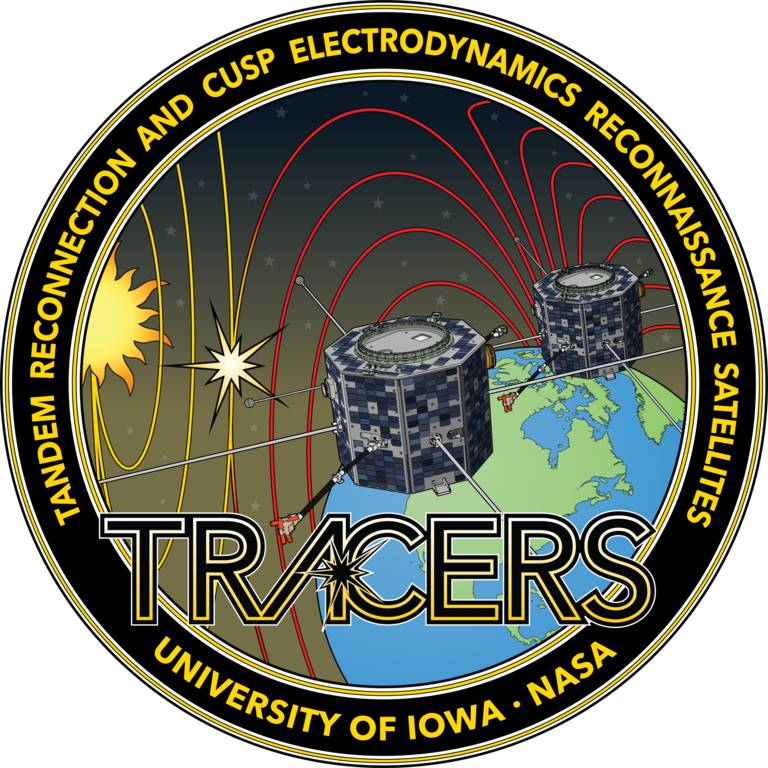